Thank you for visiting nature.com. You are using a browser version with limited support for CSS. To obtain the best experience, we recommend you use a more up to date browser (or turn off compatibility mode in Internet Explorer). In the meantime, to ensure continued support, we are displaying the site without styles and JavaScript.
Scientific Reports volume 13, Article number: 14050 (2023 ) Cite this article Polyphenol Rich Foods List

This meta-analysis aims to determine the efficacy of Epigallocatechin gallate (EGCG) in the treatment of myocardial ischemia–reperfusion injury (MIRI) and summarize the mechanisms involved. Literature from six databases including Web of Science, PubMed, Embase, China National Knowledge Infrastructure (CNKI), Wan-Fang database, and VIP database (VIP) were systematically searched. All the analysis were conducted by R. Twenty-five eligible studies involving 443 animals were included in this meta-analysis. The results indicated that compared to controls, EGCG exerts a cardioprotective effect by reducing myocardial infarct size (SMD = −4.06; 95% CI: −5.17, −2.94; P < 0.01; I2 = 77%). The funnel plot revealed publication bias. Moreover, EGCG significantly improves cardiac function, serum myocardial injury enzyme, and oxidative stress levels in MIRI animal models. This meta-analysis demonstrates that EGCG exhibits therapeutic promise in animal models of MIRI. However, further validation is still needed in large animal models and large clinical studies.
Despite substantial advances in the prevention and treatment of cardiovascular diseases (CVD), it is still the major cause of global mortality, of which the toll of death due to ischemic heart disease accounts for approximately 50%1,2,3. Acute myocardial infarction (AMI) is usually caused by prolonged ischemia of the myocardial cell4. The reperfusion treatment strategies for AMI are to open the occluded artery by thrombolytic therapy or percutaneous coronary intervention (PCI) or coronary artery bypass grafting5,6,7. Although early diagnosis and timely reperfusion are the most effective strategies to reduce myocardial ischemia injury and myocardial infarction size, reperfusion itself can also lead to myocardial cell injury and death, which is called myocardial ischemia/reperfusion injury (MIRI)8. The manifestations of MIRI mainly include ventricular arrhythmias, myocardial stunning (myocardial systolic dysfunction), microvascular obstruction (MVO), and lethal myocardial reperfusion injury9, and its pathological processes include oxidative stress, cytosolic calcium overload, rapid restoration of intracellular pH, mitochondrial dysfunction, cell apoptosis, inflammation9,10. Although numerous explorations have been made on the treatment of MIRI in recent years, no effective drug therapy has been found. Therefore, there is an urgent need to seek new effective therapeutic strategies to prevent and treat MIRI.
Green tea has been one of the most popular beverages worldwide for thousands of years, playing a pivotal role in the prevention and remission of cancer, cardiovascular disease, diabetes, and other diseases11,12. Epigallocatechin gallate (EGCG), also known as epigallocatechin-3-O-gallate, is the main component of green tea polyphenols. Previous studies have reported that EGCG confers a promising cardiovascular protective effect against cardiovascular diseases, especially MIRI, by reducing the production of reactive oxygen species (ROS), preventing intracellular Ca2+ overload13,14,15, inhibiting the inflammatory response and cell apoptosis, and promoting free radical scavenging. In addition, it can also alleviate atherosclerosis, heart failure, heart hypertrophy, arrhythmia, and other cardiovascular diseases. However, the specific efficacy and determine its underlying molecular mechanisms of EGCG in treating MIRI have not been systematically assessed to date. Therefore, we performed a meta-analysis to evaluate the cardioprotective efficacy and potential mechanisms of EGCG in MIRI animal models.
This meta-analysis has been registered in PROSPERO (ID: CRD42023390971).
We performed a systematic literature search on Web of Science, PubMed, Embase, China National Knowledge Infrastructure (CNKI), the Wan-Fang database, and the VIP database between database inception and January 2023 without language restrictions. Search items include: "myocardial I/R", "myocardial I/R injury”, “myocardial ischemia–reperfusion injury”, “myocardial ischemia–reperfusion”, “epigallocatechin-3-O-gallate”, “epigallocatechin gallate”, “epigallocatechin-3-gallate”, and “EGCG”.
Studies that met all of the following pre-established criteria were included: (1) animal models of MIRI were established by ligating the left anterior descending (LAD) coronary artery or injecting intravenously vasoconstrictor; (2) EGCG was the only intervention with a control group receiving placebo fluid or no treatment; (3) in vivo or ex vivo animal studies; (4) the primary outcome measures were myocardial infarction size, cardiac function parameters, serum myocardial enzyme and oxidative stress markers and the secondary outcome measures were mechanisms of EGCG to protect against MIRI. The pre-established exclusion criteria were as follows: (1) abstracts or meeting posters; (2) no detailed data was provided; (3) no animal model; (4) without a control group; (5) in vitro studies; (6) EGCG is not the only intervention.
Two researchers independently filtrated the title, abstract, and full text, then extracted the details of the study. When any discrepancy arose, they negotiated with correspondence authors. The research details extracted include: (1) Author information: author, publication year, and country; (2) Animal information: species, sex, and weight; (3) Animal models: anesthetic, model methods, and the number of each group; (4) Drug administration: method, dosage, and duration of administration; (5) Outcome record: the mean and standard deviation of the primary (infarct size) and secondary outcomes (cardiac function, serum myocardial injury enzyme, and oxidative stress levels). When different doses of the drug were used, data in the highest dose group was extracted. When there were multiple time points in the reperfusion time, only the last time point was recorded.
The two researchers used the SYRCLE’s RoB tool to assess and score the quality of the included studies on a 10-item scale of one point for each item, as follows: peer-reviewed publication, control of temperature, random allocation to treatment or control, blinded induction of the model, blinded evaluation of the outcome, appropriate use of anesthetic, appropriate animal model, sample size calculation, compliance with animal welfare regulations, statement of potential conflict of interests. Divergences were resolved by discussing with the corresponding author.
All statistical data in this meta-analysis were analyzed using R software (Version 4.2.2). The standard mean difference (SMD) and the 95% confidence interval (CI) were used to evaluate the effect of EGCG on myocardial infarct size, cardiac function parameters, serum myocardial enzyme, and oxidative stress markers. Heterogeneity across studies was assessed by the Cochran test and I2 statistics. It is generally believed that I2 values of 0–25%, 25–75%, and 75–100% correspond to low heterogeneity, medium heterogeneity, and high heterogeneity, respectively. The random-effects model was used to estimate the overall effect without considering heterogeneity due to different animal populations of animal-based meta-analysis. Publication bias was analyzed by funnel plots and Egger's test when more than ten studies were included in the meta-analysis. Sensitivity analysis was used to explore sources of heterogeneity. The probability values of P-value less than 0.05 are considered statistically significant.
A total of 147 potential records were retrieved from six databases, of which 29 articles were removed for reduplication. After screening titles and abstracts, 72 studies were discarded for reasons including (1) reviews, (2) case reports, (3) in vitro studies, (4) irrelevant to the topic, and (5) EGCG was not the only intervention. The full text of the remaining studies was examined, of which 21 were excluded for the following reasons: (1) no available data, (2) no MIRI model, (3) meeting abstracts or patents, and (4) duplication. Finally, 25 eligible studies were included3,14,16,17,18,19,20,21,22,23,24,25,26,27,28,29,30,31,32,33,34,35,36,37,38,39. The PRISMA flow diagram of literature screening in this study is shown in Fig. 1.
Flowchart for database search and study selection.
A total of 25 studies with 443 animals (221 in the EGCG-treated group and 222 in the control group) were included between 2004 and 2021. In terms of countries, 12 of the 26 studies were conducted in China, five studies in Korea, two each in India and Japan, and one each in the United Kingdom, the United States, Egypt, and Germany. Wistar rats were used in nine studies, SD rats were used in 12 studies, C57BL/6 mice were used in two studies, and Hartley strain guinea pigs and Chinchilla Bastard rabbits were used in one study. A total of 22 studies established the MIRI model by LAD ligation, of which eight studies were by LAD occlusion in isolated perfused hearts with Langendorff, and three studies established MIRI by intravenous injection of isoprenaline (ISO). EGCG was administered intravenously in nine studies, intraperitoneally in one study, orally in seven studies, and perfused to the heart in eight studies. The characteristics of the 25 enrolled studies are presented in Table 1.
The quality score of the included studies ranged from 3 to 7. Three studies scored 4 points, nine studies scored 5 points, and 11 studies scored 6 points. The remaining two studies scored 3 and 7, respectively. All studies were published in peer-reviewed journals. All animals were randomly allocated to the treatment or control groups with appropriate animal models (aged, diabetic, or hypertensive). However, no study describes the blinded induction of the model and sample size calculation. Blinded assessment of outcome was described in two studies. Nine studies mentioned control of temperature. A total of 21 studies used anesthetic without significant intrinsic cardioprotective activity. Animals in 21 studies complied with animal welfare regulations. Only five studies included a statement of potential conflict of interest. The methodological quality of the included studies is shown in Table 2. The molecular mechanism of included studies that EGCG protects cardiomyocytes from MIRI was summarized in Table 3.
A meta-analysis of 15 studies, involving 231 animals19,21,22,23,25,27,28,30,31,32,33,34,35,36,37, showed that the EGCG treatment significantly reduced the myocardial infarct size (IS) compared to controls (SMD = −4.06; 95% CI: −5.17, −2.94; P < 0.01; I2 = 77%) (Fig. 2a). Due to the apparent heterogeneity of the included publications, we performed a funnel plot and sensitivity analysis. The funnel plot was asymmetrical and the P-value of Egger's test and Begg's test was less than 0.05, indicating publication bias (Fig. 2b). The sensitivity analysis did not reveal any heterogeneous source of IS. This supported the result of IS was stable and reliable (Fig. 2c).
Forest plot displaying the protective effect of EGCG on infarct size in an animal model of myocardial ischemia/reperfusion injury (A); funnel plot assessing publication bias of infarct size in included studies (B); sensitivity analysis of infarct size (C). EGCG epigallocatechin gallate.
We conducted subgroup analysis to explore the sources of heterogeneity further. As shown in Table 4, there are significant differences in the analysis between subgroups of species, drug delivery method, and duration, which may be an important source of heterogeneity.
Five markers, consisting of left ventricular developed pressure (LVDP), left ventricular end-diastolic pressure (LVEDP), left ventricular systolic pressure (LVSP), + dP/dT max, and −dP/dt max, were analyzed to reveal the improvement effect of EGCG on cardiac function in MIRI animals. Meta-analysis of three studies with 51 animals indicated that compared to controls EGCG has a potential effect on decreasing LVDP (SMD = 3.12; 95% CI: −0.01, 6.24; P = 0.05; I2 = 86%; Fig. 3a)14,21,38. Altogether, five studies of 84 animals showed that EGCG significantly reduced LVEDP (SMD = −5.33; 95% CI: −7.70, −2.96; P < 0.01; I2 = 76%; Fig. 3b)14,20,27,35,39. A meta-analysis of 4 studies involving 60 animals found that EGCG marked increased LVSP (SMD = 5.30; 95% CI: 4.08, 6.52; P < 0.01; I2 = 0%; Fig. 3c)20,33,35,39. A total of seven studies involving 113 animals used + dP/dt max and − dP/dt max as outcome indicators, and the analysis results showed that EGCG treatment significantly improved + dP/dt max (SMD = 4.30; 95% CI: 2.49, 6.11; P < 0.01; I2 = 88%; Fig. 3d) and − dP/dt max (SMD = 3.89; 95% CI: 1.40, 6.38; P < 0.01; I2 = 88%; Fig. 3e)20,21,29,33,35,38,39.
Forest plot illustrating the cardioprotective effect of EGCG on myocardial ischemia/reperfusion injury animal models of LVDP (A), LVEDP (B), LVSP (C), + dP/dt max (D), − dP/dt max (E). LVDP left ventricular developed pressure, LVEDP left ventricular end-diastolic pressure, LVSP left ventricular systolic pressure, dP/dt max maximum, 1st derivative of developed pressure, EGCG epigallocatechin gallate.
The effects of EGCG on serum biomarkers of myocardial injury were evaluated by creatine kinase (CK), creatine kinase isoenzyme (CK-MB), lactic dehydrogenase (LDH), and troponin T (TnT). 4 publications involved 64 animals utilizing CK16,17,20,28, six publications involved 112 animals utilizing CK-MB16,24,26,34,35,39, eight publications involved 132 animals utilizing LDH16,20,26,27,28,33,35,39, and two publications involved 24 animals utilizing TnT26,32, as the outcome measure. Compared to controls, EGCG significantly reduces serum CK (SMD = −4.66; 95% CI: −6.72, −2.59; P < 0.01; I2 = 73%; Fig. 4a), CK-MB (SMD = −6.77; 95% CI: −9.31, −4.24; P < 0.01; I2 = 77%; Fig. 4b), LDH (SMD = −5.06; 95% CI: −7.17, −2.95; P < 0.01; I2 = 80%; Fig. 4c), and TnT (SMD = −9.76; 95% CI: −15.36, −4.16; P < 0.01; I2 = 57%; Fig. 4d) in MIRI animals.
Forest plot showing cardioprotective effects of EGCG on CK (A), CK-MB (B), LDH (C), and TnT (D) in an animal model of myocardial ischemia/reperfusion injury. CK creatine kinase, CK-MB creatine kinase isoenzyme, LDH lactate dehydrogenase, TnT troponin T, EGCG epigallocatechin gallate.
To evaluate the antioxidant capacity of EGCG in MIRI animal models, malondialdehyde (MDA), superoxide dismutase (SOD), and catalase (CAT) were analyzed. 5 studies with a total of 82 animals for SOD16,18,19,20,26, three studies with 50 animals for MDA19,20,26, and three studies with 44 animals for CAT16,18,26 were included. As compared to controls, EGCG significantly attenuated oxidative stress by increasing serum SOD (SMD = 4.30; 95% CI: 3.40, 5.20; P < 0.01; I2 = 51%; Fig. 5a), decreasing MDA (SMD = −7.63; 95% CI: −10.38, −4.87; P < 0.01; I2 = 54%; Fig. 5b), and increasing CAT (SMD = 13.33; 95% CI: 4.89, 21.76; P < 0.01; I2 = 85%; Fig. 5c).
Forest plot illustrating the cardioprotective effects of EGCG on SOD (A), MDA (B), and CAT (C) in an animal model of myocardial ischemia/reperfusion injury. SOD superoxide dismutase, MDA malondialdehyde, CAT catalase.
We conduct the first meta-analysis of preclinical studies to assess the cardioprotective efficacy of EGCG in MIRI. A total of 25 studies with 443 animals were included. The evidence available in our study indicated that EGCG exerted a cardioprotective role by reducing myocardial IS and the expression of serum myocardial enzymes, improving cardiac function parameters, and reducing oxidative stress level.
Numerous studies have shown that MIRI is closely associated with various pathological processes such as inflammation, oxidation stress, cardiomyocyte apoptosis, autophagy, etc40. An in-depth understanding of the underlying mechanisms of EGCG will serve to better understand the cardioprotective effects of EGCG. Therefore, we summarize the potential mechanisms involved in the myocardial protective effects of EGCG as follows:
During MIRI, necrotic cardiomyocytes activate inflammatory responses and exacerbate myocardial injury by inducing oxidative stress, triggering the complement cascade, and releasing danger-associated molecular patterns (DAMPs). Myeloperoxidase (MPO) is a neutrophil-specific heme peroxidase that is closely related to the inflammatory process of ischemia–reperfusion injury41. EGCG plays an anti-inflammatory role by reducing MPO activity and the infiltration of neutrophils17.
Mitochondrial DNA (mtDNA), a naked circular or linear DNA, has been identified as a damage-associated molecular pattern (DAMP) that can trigger a series of inflammatory responses42,43. In MIRI, the expression of mtDNA was positively correlated with the expression of TNF-a, IL-6, and IL-844. The PI3K/Akt pathway reduces myocardial injury by negatively regulating the inflammatory response45. The activation of the PI3K/Akt pathway is a critical pathway of cardioprotective and is associated with preservation of mitochondrial function during MIRI46. EGCG inhibits inflammation response to reduce myocardial IS by activating the PI3K/Akt pathway to reduce the release of pro-inflammatory mediator mtDNA and the secretion of inflammatory factors in plasma28. In addition, EGCG exerts anti-inflammatory effects and protects cardiomyocytes by inhibiting IKK/NF-kB and c-Jun/AP-1 pathways17.
Oxidative stress caused by increased reactive oxygen species (ROS) is one of the main pathological mechanisms for the occurrence and development of MIRI. Due to the presence of multiple phenolic hydroxyl groups that are easily oxidized to quinones in the structure, EGCG exerts powerful antioxidant properties in the treatment of MIRI by regulating the redox system to scavenge free radicals and inhibiting lipid peroxidation, thereby alleviating myocardial damage47,48. Specifically, EGCG plays an antioxidant effect by significantly increasing the endogenous antioxidant activity of antioxidant enzymes (SOD, CAT, GPx, GRx, GST) and antioxidants (vitamin C, vitamin E, ceruloplasmin), and inhibiting the accumulation of MDA, thiobarbituric acid reactive substances, and uric acid26,49.
SIRT1 (Sirtuin 1) is a nicotinamide adenine dinucleotide (NAD)-dependent histone deacetylase involved in regulating oxidative stress resistance under ischemic and hypoxic conditions50. In diabetic MIRI rats, EGCG increases the expression of the antioxidant enzyme manganese superoxide dismutase (MnSOD) by activating SIRT1 and reducing oxidative stress damage33. Calcium overload is a major cause of cellular damage during MIRI51. Activation of KATP channels protects cardiomyocytes from MIRI-induced Ca2+ overload52. It has been found that the reduction in infarct size produced by myocardial ischemic preconditioning and pre-ischemic drug therapy is partly attributed to mitochondrial potassium ATP (mKATP) channels53,54. In addition, in Ossabaw minipigs, activating KATP channels by the ischemic preconditioning (IPC) process can delay the magnitude of ST-segment elevation55, further suggesting that opening mitochondrial KATP channels can play a cardioprotective role. During myocardial ischemia in isolated mice hearts, EGCG opens mKATP channels by activating A1 and A2B adenosine receptors to relieve Ca+ overload pressure and increase NAD+ expression, reduce the opening time of mitochondrial permeability transition (mPTP) pore and finally reduce the amount of ROS14,23,30,56.
Apoptosis, as one of the critical mechanisms in the MIRI process, plays a dual role in MIRI according to the degree of apoptosis. On the one hand, proper apoptosis reduces myocardial cell necrosis and plays a protective role; on the other hand, excessive apoptosis aggravates myocardial injury and accelerates myocardial cell death.
In the MIRI model, EGCG alleviated cardiomyocyte apoptosis/exerts antiapoptotic effects by up-regulating the expression of anti-apoptotic protein Bcl-2, down-regulating the expression of pro-apoptotic proteins p53, Bax, cleaved-caspase-3 and caspase-926,27. Existing evidence has identified the PI3K/Akt signaling pathway played a protective role in protecting cardiomyocytes from the two aspects of promoting the survival of cardiomyocytes and inhibiting the apoptosis of cardiomyocytes by regulating the expression of apoptotic proteins and transcription factors57. In MIRI models, EGCG increases the survival rate of cardiomyocytes by activating PI3K/Akt signaling pathway, promoting the phosphorylation of eNOS, and increasing the NO content35. JNK and p38 are members of the mitogen-activated protein kinase (MAPK) family, and the JNK/p38 MAPK cascade plays a pivotal role in mediating apoptosis. EGCG inhibits p38 and JNK phosphorylation, decreases Caspase-3 expression, plays an antiapoptotic role in cardiomyocytes, and reduces the size of myocardial infarction22,38. STAT-1 is a signal transducer and transcriptional activator, and its phosphorylation can induce apoptosis by mediating the expression of apoptosis-related genes58. EGCG is an effective inhibitor of STAT1 phosphorylation, which can reduce the expression of caspase-3 and the degree of myocardial cell apoptosis by inhibiting the activation of the STAT-1/Fas pathway59. miR-30, a member of the MicroRNA family, regulates cell apoptosis by targeting the inhibition of the mitochondrial apoptosis activator p5360. In hypoxia-reoxygenation (H/R)-induced H9C2 cells and I/R-induced rats, EGCG can inhibit mPTP opening and anti-apoptotic protein expression by activating the miR-30a/p53 signaling pathway15.
Autophagy plays opposite roles in different periods of myocardial injury. Autophagy in ischemia can supply energy and play a role in cardiac protection, while in reperfusion its excessive activation accelerates cardiomyocyte death61. During ischemia, EGCG trigger autophagy to protect cells from apoptosis by regulating mTOR negative feedback mechanism24. During reperfusion, EGCG protects against MIRI by activating PI3K/Akt/mTOR signaling cascade, reducing Beclin-1 expression, and restoring autophagy flux to inhibit excessive autophagy24,35. Recent studies have shown that long non-coding RNA GM4419 can alleviate myocardial infarction by activating miR-682/TRF3 in I/R and H/R-induced myocardial injury models62. EGCG reduces the degree of myocardial injury by reducing Gm4419 expression and epigenetic silencing/inhibiting DUSP5/ERK1/2 signaling pathway-mediated autophagy in H2O2-induced cardiomyocytes and I/R-induced mouse models63. ATG4C, a cysteine enzyme, is involved in autophagy by regulating the functions of LC3 and ATG864,65. EGCG can increase ATG4C expression and decrease LC3II expression, thus inhibiting H/R-induced apoptosis and autophagy of H9C2 cells66. In atherosclerotic diseases, miR-384 has been found to target and inhibit Beclin-1 to suppress macrophage autophagy27. In MIRI, EGCG can inhibit autophagy and alleviate the injury by activating PI3K/Akt pathway, increasing miR-384, and decreasing Beclin137. EGCG can exert cardioprotective effects from three aspects of anti-inflammation, anti-apoptosis, and autophagy by regulating the PI3K/Akt pathway, suggesting an important role of the PI3K/Akt pathway in the cardioprotective process of EGCG.
During myocardial infarction, platelets play a dual role of promoting arterial thrombosis leading to cardiac injury and regulating cardiomyocyte secretion of factors leading to cardioprotection67,68. It has been found that EGCG can inhibit platelet aggregation induced by U46619, collagen, arachidonic acid, and toxic carotenoids and shear force-induced platelet adhesion dose-dependently by suppressing PLCγ2 and tyrosine phosphorylation of various platelet proteins, up-regulating the expression of intracellular PGD2, blocking the increase of intracytoplasmic free calcium ions and reducing the release of arachidonic acid (AA), thus delaying the formation of arterial thrombus and exerting potent antithrombotic effects. What’s more, its combination with common antiplatelet therapeutic agents, aspirin (ASA), clopidogrel (CPD), and tiglitazarol (TCG), did not further inhibit platelet aggregation resulting in bleeding complications, demonstrating the potent antiplatelet effect of EGCG and its favorable safety profile. The antiplatelet and thrombotic activities of EGCG may be partially attributed to the presence of the galloyl group at the 3' position of C ring69,70,71. In addition, EGCG inhibits platelet activation by inhibiting microsomal cyclooxygenase-1 activity in platelets as well as platelet extracellular vesicle release72,73. During MIRI, platelets can exacerbate ischemia/reperfusion (IR) injury by promoting thrombosis, decreasing myocardial perfusion, secreting vasoconstrictors, and causing endothelial dysfunction67,68. Therefore, we speculated that EGCG may exert cardioprotective effects during MIRI by inhibiting platelet aggregation and thrombosis, but further experimental validation is needed.
Myocardium ischemia–reperfusion inevitably leads to myocardial cell and coronary microvascular injury. Among them, coronary microvascular injury includes various mechanisms such as coronary microembolism (CME), platelet activation, endothelial dysfunction, and increased permeability, which eventually lead to capillary injury, complications of no-reflow, intramyocardial hemorrhage, and adverse microvascular obstruction, which has been identified as one of the key factors affecting the prognosis of patients with acute myocardial infarction74,75,76. Coronary microcirculation has increasingly become an effective target for cardioprotection during the treatment of acute myocardial infarction. Cardiomyocyte apoptosis and myocardial inflammation induced by coronary microembolism, a common complication during ACS and PCI treatment, are considered to be one of the main mechanisms of myocardial injury and cardiac dysfunction77. Inhibition of cardiomyocyte apoptosis and myocardial inflammation attenuated CME-induced myocardial injury and improved cardiac dysfunction78. Although there is no report of EGCG improving coronary microcirculation by treating coronary microembolism, other flavonoids such as curcumin (CCM) have been shown to inhibit CME-induced myocardial inflammation and cardiomyocyte apoptosis through the TLR4/MyD88/NF-kβ signaling axis79, implying that EGCG may also have the potential of improving coronary microcirculation, but the specific research still needs to be further studied. In addition, a study based on four different structures of theaflavins (TFs) found that chemicals containing only a single galloyl group improved coronary microcirculation, while none or two galloyl groups chemicals were ineffective80. Since the chemical structure of EGCG happens to contain a single galloyl group, we speculate that EGCG may also have the effect of improving coronary microcirculation. In addition, two clinical trials have found that EGCG supplementation improves endothelial dysfunction in the short term during the treatment of patients with coronary artery disease81. Therefore, we speculated that EGCG might also have the capability to improve coronary microcirculation according to both the effects of its structural analogs as well as its structural properties, but it remains to be further verified experimentally.
Although there is currently a dearth of clinical trials on the cardioprotective effects of EGCG against MIRI, several clinical trial studies have reported that EGCG protects against multiple aspects of a wide range of cardiovascular diseases. For example, two clinical studies found that EGCG reduced the risk of cardiovascular disease in obese subjects by significantly lowering plasma triglyceride levels, as well as blood pressure82,83. In normal male healthy volunteers, EGCG may delay the progression of oxidative stress-associated atherosclerotic disease by decreasing low-density lipoprotein (LDL) oxidizing capacity84. A clinical trial based on patients with mild to moderate hypertension also discovered that the administration of Benifuuki, whose active ingredient is an EGCG-O-methylated derivative, exerts a hypotensive effect by significantly inhibiting angiotensin I-converting enzyme activity85. Furthermore, in patients with amyloid transthyretin cardiomyopathy, EGCG may curb cardiac amyloidosis by reducing left ventricular myocardial mass86,87. In terms of anti-platelet drug therapy, EGCG was found to reduce adenosine diphosphate (ADP)- and collagen (COL)-induced platelet aggregation, as well as shear force-induced platelet adhesion dose-dependently, and its combination with common antiplatelet therapeutic agents, aspirin (ASA), clopidogrel (CPD), and tiglitazarol (TCG), did not further inhibit platelet aggregation resulting in bleeding complications, demonstrating the potent antiplatelet effect of EGCG and its favorable safety profile71. As for the effects of EGCG on endothelial dysfunction, clinical trials based on healthy subjects have shown that EGCG has no effect on improving endothelial dysfunction88; whereas, in patients with atherosclerosis, EGCG can reduce the incidence of cardiovascular disease by improving endothelial function89; and in patients with coronary artery disease, the acute administration of EGCG significantly reversed endothelial dysfunction in coronary patients in the short term. The ability of EGCG to improve endothelial dysfunction may be related to the subjects studied in the clinical trials, and EGCG may be effective in improving endothelial dysfunction in patients with cardiovascular disease in the short term, but not in healthy subjects, and the specific efficacy of EGCG remains to be further verified by trials in the future. The above clinical trial results suggest that EGCG may exert cardioprotective effects through multiple mechanisms, including lowering blood pressure, improving endothelial dysfunction, and inhibiting platelet aggregation and adhesion.
Reperfusion injury will inevitably lead to myocardial cell death and cardiac dysfunction during the treatment of acute myocardial infarction90, but until now there is no clinical intervention drug to improve MIRI91, so it is urgent to seek a new potential therapeutic drug to improve MIRI. Studies reported that EGCG reduces myocardial injury by reducing oxidative stress, inhibiting apoptosis and inflammatory response, and regulating autophagy and mitochondrial function during MIRI47. The results of this study showed that EGCG significantly reduces myocardial infarct size, improves cardiac function, down-regulates myocardial enzyme levels, and inhibits oxidative stress to play a cardioprotective role in MIRI animal models.
Studies have shown that meta-analysis and systematic review to evaluate the therapeutic efficacy of drugs for experimental animal research can help the translation of research results from animal experiments to clinical applications and narrow the gap between the two92. During the study inclusion process, we considered the relevance of the article to the research topic during the title and abstract selection process and the full-text review process and evaluated the quality of the article according to 10 criteria. These steps contributed to improving the stability and reliability of the results of this meta-analysis. However, the results of this study only used youthful and healthy small animal models and did not include aged small animal models with multiple coexisting diseases such as diabetes and hypertension as well as coadministration of medications, which may differ from the complex pathology of patients in the actual clinical setting. According to Improving the Preclinical Assessment of Cardioprotective Therapies (IMPACT) guidelines in the EU Cardiac Protection Cost Action Guidelines, the first step in reducing the risk of failure in the translation of preclinical research into clinical research requires the use of healthy, young animals for initial experiments, but in the future, small animal models with multiple confounding factors still need to be further verified93. This study only included young and healthy small animal models, so the actual efficacy of EGCG still needs to be further verified in small animal models with at least one confounding factor. In addition, the results of this study were not validated in large animal models, which are crucial for the translation of preclinical research to clinical research, because the anatomy and vascular dynamics of large animals, especially pigs, are much closer to the actual configuration of human beings so that the clinical model can better mimic that of MIRI under specific conditions, which can aide in clinical translation. Moreover, animal research itself has certain methodological flaws, design variations, and publication bias caused by the fact that negative results are more difficult to be published, further widening the gap between animal experiments and clinical applications94. Nevertheless, to further facilitate the translation of EGCG from animal studies to clinical practice, more small animal models with multiple confounding factors, high-quality large-scale animal studies, and randomized controlled trials are still needed for further discussion and validation.
First, we only retrieved studies in Chinese and English databases, lacking studies in other language databases, which may cause a certain degree of selection bias. Secondly, negative results from drug studies are less likely to be published, which may lead to an overestimation of drug efficacy. Thirdly, all the animals included in the study did not adopt the disease model of myocardial injury and related comorbidities, such as advanced age, hypertension, hyperlipidemia, diabetes, etc., while patients with clinical myocardial injury often suffer from multiple diseases.
This meta-analysis demonstrates that EGCG exhibits therapeutic promise in animal models of MIRI. However, further validation is still needed in large animal models and large clinical studies.
The data used to support the findings of this study are included in the article. Further inquiries can be directed to the corresponding authors.
Article PubMed PubMed Central Google Scholar
Roth, G. A. et al. Global burden of cardiovascular diseases and risk factors, 1990–2019: Update from the GBD 2019 study. J. Am. Coll. Cardiol. 76(25), 2982–3021 (2020).
Article PubMed PubMed Central Google Scholar
Winter, S. J., Sheats, J. L. & King, A. C. The use of behavior change techniques and theory in technologies for cardiovascular disease prevention and treatment in adults: A comprehensive review. Prog. Cardiovasc. Dis. 58(6), 605–612 (2016).
Article PubMed PubMed Central Google Scholar
Thygesen, K. et al. Fourth universal definition of myocardial infarction (2018). J. Am. Coll. Cardiol. 72(18), 2231–2264 (2018).
Doenst, T. et al. PCI and CABG for treating stable coronary artery disease: JACC review topic of the week. J. Am. Coll. Cardiol. 73(8), 964–976 (2019).
Sabatine, M. S. & Braunwald, E. Thrombolysis In Myocardial Infarction (TIMI) Study Group: JACC focus seminar 2/8. J. Am. Coll. Cardiol. 77(22), 2822–2845 (2021).
Mackman, N. et al. Therapeutic strategies for thrombosis: New targets and approaches. Nat. Rev. Drug Discov. 19(5), 333–352 (2020).
Article CAS PubMed Google Scholar
Heusch, G. & Gersh, B. J. The pathophysiology of acute myocardial infarction and strategies of protection beyond reperfusion: A continual challenge. Eur. Heart J. 38(11), 774–784 (2017).
Hausenloy, D. J. & Yellon, D. M. Myocardial ischemia–reperfusion injury: A neglected therapeutic target. J. Clin. Invest. 123(1), 92–100 (2013).
Article CAS PubMed PubMed Central Google Scholar
Golforoush, P., Yellon, D. M. & Davidson, S. M. Mouse models of atherosclerosis and their suitability for the study of myocardial infarction. Basic Res. Cardiol. 115(6), 73 (2020).
Article PubMed PubMed Central Google Scholar
Pae, M. & Wu, D. Immunomodulating effects of epigallocatechin-3-gallate from green tea: Mechanisms and applications. Food Funct. 4(9), 1287–1303 (2013).
Article CAS PubMed Google Scholar
Zeng, X. et al. Green tea may be benefit to the therapy of atrial fibrillation. J. Cell Biochem. 112(7), 1709–1712 (2011).
Article CAS PubMed Google Scholar
Liou, Y. M. et al. Green tea extract given before regional myocardial ischemia–reperfusion in rats improves myocardial contractility by attenuating calcium overload. Pflugers Arch. 460(6), 1003–1014 (2010).
Article CAS PubMed Google Scholar
Hirai, M. et al. Protective effects of EGCg or GCg, a green tea catechin epimer, against postischemic myocardial dysfunction in guinea-pig hearts. Life Sci. 80(11), 1020–1032 (2007).
Article CAS PubMed Google Scholar
Zhang, C. et al. Epigallocatechin gallate prevents mitochondrial impairment and cell apoptosis by regulating miR-30a/p53 axis. Phytomedicine 61, 152845 (2019).
Article CAS PubMed Google Scholar
Devika, P. T. & Prince, P. S. M. (−)Epigallocatechin gallate (EGCG) prevents isoprenaline-induced cardiac toxicity by stabilizing cardiac marker enzymes and membrane-bound ATPases. J. Pharm. Pharmacol. 60(1), 125–133 (2008).
Article CAS PubMed Google Scholar
Aneja, R. et al. Epigallocatechin, a green tea polyphenol, attenuates myocardial ischemia reperfusion injury in rats. Mol. Med. 10(1–6), 55–62 (2004).
Article CAS PubMed PubMed Central Google Scholar
Devika, P. T. & Prince, P. S. M. Protective effect of (−)-epigallocatechin-gallate (EGCG) on lipid peroxide metabolism in isoproterenol induced myocardial infarction in male Wistar rats: A histopathological study. Biomed. Pharmacother. 62(10), 701–708 (2008).
Article CAS PubMed Google Scholar
Fu, W. et al. Study on mechanism of epigallocatechin gallate alleviating myocardial ischemia–reperfusion injury by inhibiting cardiomyocyte apoptosis. China Pharm. 30(16), 2187–2192 (2019).
Hu, Z. The protective role of epigallocatechin gallate on myocardial ischemia reperfusion injury in rats. Chin. J. Mod. Appl. Pharm. 06, 442–445 (2005).
Kim, C. J. et al. Polyphenol (−)-epigallocatechin gallate targeting myocardial reperfusion limits infarct size and improves cardiac function. Korean J. Anesthesiol. 58(2), 169–175 (2010).
Article MathSciNet CAS PubMed PubMed Central Google Scholar
Kim, S. J. et al. Epigallocatechin-3-gallate, a green tea catechin, protects the heart against regional ischemia–reperfusion injuries through activation of RISK survival pathways in rats. Arch. Pharm. Res. 37(8), 1079–1085 (2014).
Article CAS PubMed Google Scholar
Lee, S. K. et al. Polyphenol (−)-epigallocatechin gallate-induced cardioprotection may attenuate ischemia–reperfusion injury through adenosine receptor activation: A preliminary study. Korean J. Anesthesiol. 63(4), 340–345 (2012).
Article CAS PubMed PubMed Central Google Scholar
Li, C., Sun, Y. & Jian, J. Epigallocatechin gallate alleviates myocardial ischemia reperfusion injury by adjusting autophagy. Chin. J. New Drugs Clin. Remed. 34(06), 471–475 (2015).
Lin, CM et al.(−)-Epigallocatechin gallate promotes microRNA 145 expression against myocardial hypoxic injury through Dab2/Wnt3a/β-catenin.Am.J. Chin.Med.48(2), 341–356 (2020).
Othman, A. I. et al. Epigallocatechin-3-gallate prevents cardiac apoptosis by modulating the intrinsic apoptotic pathway in isoproterenol-induced myocardial infarction. Eur. J. Pharmacol. 794, 27–36 (2017).
Article CAS PubMed Google Scholar
Piao, C. S. et al. The protective effect of epigallocatechin-3 gallate on ischemia/reperfusion injury in isolated rat hearts: An ex vivo approach. Korean J. Physiol. Pharmacol. 15(5), 259–266 (2011).
Article CAS PubMed PubMed Central Google Scholar
Qin, C.-Y. et al. Mitochondrial DNA-induced inflammatory damage contributes to myocardial ischemia reperfusion injury in rats: Cardioprotective role of epigallocatechin. Mol. Med. Rep. 16(5), 7569–7576 (2017).
Article CAS PubMed Google Scholar
Salameh, A. et al. Epigallocatechin gallate reduces ischemia/reperfusion injury in isolated perfused rabbit hearts. Int. J. Mol. Sci. 19(2), 628 (2018).
Song, D.-K. et al. Polyphenol (−)-epigallocatechin gallate during ischemia limits infarct size via mitochondrial K(ATP) channel activation in isolated rat hearts. J. Korean Med. Sci. 25(3), 380–386 (2010).
Article CAS PubMed PubMed Central Google Scholar
Townsend, P.A. et al. Epigallocatechin-3-gallate inhibits STAT-1 activation and protects cardiac myocytes from ischemia/reperfusion-induced apoptosis. Faseb J. 18(11), 1612-+ (2004).
Tu, Q. et al. EGCG decreases myocardial infarction in both I/R and MIRI rats through reducing intracellular Ca2+ and increasing TnT levels in cardiomyocytes. Adv. Clin. Exp. Med. 30(6) (2021).
Wu, Y. et al. (−)-Epigallocatechin-3-gallate attenuates myocardial injury induced by ischemia/reperfusion in diabetic rats and in H9c2 cells under hyperglycemic conditions. Int. J. Mol. Med. 40(2), 389–399 (2017).
Article CAS PubMed PubMed Central Google Scholar
Xin, S. et al. Protective effect of epigallocatechin-3-gallate against myocardial ischemia and reperfusion injury in rats. Chin. J. Primary Med. Pharm. 08, 1309–1311+1410 (2008).
Xuan, F. & Jian, J. Epigallocatechin gallate exerts protective effects against myocardial ischemia/reperfusion injury through the PI3K/Akt pathway-mediated inhibition of apoptosis and the restoration of the autophagic flux. Int. J. Mol. Med. 38(1), 328–336 (2016).
Article CAS PubMed Google Scholar
Zeng M. et al. EGCG protects against myocardial I/RI by regulating lncRNA Gm4419-mediated epigenetic silencing of the DUSP5/ERK1/2 axis. Toxicol. Appl. Pharmacol. 433 (2021).
Zhang, C. et al. MicroRNA-384-5p/beclin-1 as potential indicators for epigallocatechin gallate against cardiomyocytes ischemia reperfusion injury by inhibiting autophagy via PI3K/Akt pathway. Drug Des. Dev. Ther. 13, 3607–3623 (2019).
Article ADS CAS Google Scholar
Yanagi, S. et al. Oral pretreatment with a green tea polyphenol for cardioprotection against ischemia–reperfusion injury in an isolated rat heart model. J. Thorac. Cardiovasc. Surg. 141(2), 511–517 (2011).
Article CAS PubMed Google Scholar
Zhang, C. et al. Epigallocatechin gallate prevents mitochondrial impairment and cell apoptosis by regulating miR-30a/p53 axis. Phytomedicine 61, 152845 (2019).
Schirone, L. et al. An overview of the molecular mechanisms associated with myocardial ischemic injury: State of the art and translational perspectives. Cells 11(7), 1165 (2022).
Ndrepepa, G. Myeloperoxidase—A bridge linking inflammation and oxidative stress with cardiovascular disease. Clin. Chim. Acta 493, 36–51 (2019).
Article CAS PubMed Google Scholar
Zhang, Q. et al. Circulating mitochondrial DAMPs cause inflammatory responses to injury. Nature 464(7285), 104–107 (2010).
Article ADS CAS PubMed PubMed Central Google Scholar
Yue, R. et al. Mitochondrial DNA oxidative damage contributes to cardiomyocyte ischemia/reperfusion-injury in rats: Cardioprotective role of lycopene. J. Cell Physiol. 230(9), 2128–2141 (2015).
Article CAS PubMed Google Scholar
Qin, C. et al. Variation of perioperative plasma mitochondrial DNA correlate with peak inflammatory cytokines caused by cardiac surgery with cardiopulmonary bypass. J. Cardiothorac. Surg. 10, 85 (2015).
Article PubMed PubMed Central Google Scholar
Williams, D. L., Ozment-Skelton, T. & Li, C. Modulation of the phosphoinositide 3-kinase signaling pathway alters host response to sepsis, inflammation, and ischemia/reperfusion injury. Shock 25(5), 432–439 (2006).
Article CAS PubMed Google Scholar
Ghafouri-Fard, S. et al. Interplay between PI3K/AKT pathway and heart disorders. Mol. Biol. Rep. 49(10), 9767–9781 (2022).
Article CAS PubMed PubMed Central Google Scholar
Singh, B. N., Shankar, S. & Srivastava, R. K. Green tea catechin, epigallocatechin-3-gallate (EGCG): Mechanisms, perspectives and clinical applications. Biochem. Pharmacol. 82(12), 1807–1821 (2011).
Article CAS PubMed PubMed Central Google Scholar
Devika, P. T. & Prince, P. S. Preventive effect of (−)epigallocatechin-gallate (EGCG) on lysosomal enzymes in heart and subcellular fractions in isoproterenol-induced myocardial infarcted Wistar rats. Chem. Biol. Interact. 172(3), 245–252 (2008).
Article CAS PubMed Google Scholar
Devika, P.T., & Stanely Mainzen Prince, P. Protective effect of (−)-epigallocatechin-gallate (EGCG) on lipid peroxide metabolism in isoproterenol induced myocardial infarction in male Wistar rats: A histopathological study. Biomed. Pharmacother. 62(10), 701–708 (2008).
Yang, Y. et al. Novel role of silent information regulator 1 in myocardial ischemia. Circulation 128(20), 2232–2240 (2013).
Wang, L. et al. Preconditioning limits mitochondrial Ca(2+) during ischemia in rat hearts: Role of K(ATP) channels. Am. J. Physiol. Heart Circ. Physiol. 280(5), H2321–H2328 (2001).
Article CAS PubMed Google Scholar
Ylitalo, K. V. et al. Intracellular free calcium and mitochondrial membrane potential in ischemia/reperfusion and preconditioning. J. Mol. Cell Cardiol. 32(7), 1223–1238 (2000).
Article CAS PubMed Google Scholar
Garlid, K. D. et al. Cardioprotective effect of diazoxide and its interaction with mitochondrial ATP-sensitive K+ channels. Possible mechanism of cardioprotection. Circ. Res. 81(6), 1072–1082 (1997).
Article CAS PubMed Google Scholar
O’Rourke, B. Myocardial K(ATP) channels in preconditioning. Circ. Res. 87(10), 845–855 (2000).
Article CAS PubMed Google Scholar
Lieder, H. R. et al. Attenuation of ST-segment elevation by ischemic preconditioning: Reflection of cardioprotection in Göttingen but not in Ossabaw minipigs. Int. J. Cardiol. 386, 109–117 (2023).
Tu, Q. et al. EGCG decreases myocardial infarction in both I/R and MIRI rats through reducing intracellular Ca2+ and increasing TnT levels in cardiomyocytes. Adv. Clin. Exp. Med. 30(6), 607–616 (2021).
Ha, T. et al. TLR2 ligands induce cardioprotection against ischaemia/reperfusion injury through a PI3K/Akt-dependent mechanism. Cardiovasc. Res. 87(4), 694–703 (2010).
Article CAS PubMed PubMed Central Google Scholar
Stephanou, A. & Latchman, D. S. STAT-1: A novel regulator of apoptosis. Int. J. Exp. Pathol. 84(6), 239–244 (2003).
Article CAS PubMed PubMed Central Google Scholar
Townsend, P. A. et al. Epigallocatechin-3-gallate inhibits STAT-1 activation and protects cardiac myocytes from ischemia/reperfusion-induced apoptosis. Faseb J. 18(13), 1621–1623 (2004).
Article CAS PubMed Google Scholar
Ferenczyová, K. et al. Pharmacology of catechins in ischemia–reperfusion injury of the heart. Antioxidants (Basel) 10(9), 1390 (2021).
Schiattarella, G. G. & Hill, J. A. Therapeutic targeting of autophagy in cardiovascular disease. J. Mol. Cell Cardiol. 95, 86–93 (2016).
Article CAS PubMed Google Scholar
Tao, S. et al. Linc00514 promotes breast cancer metastasis and M2 polarization of tumor-associated macrophages via Jagged1-mediated notch signaling pathway. J. Exp. Clin. Cancer Res. 39(1), 191 (2020).
Zeng, M. et al. EGCG protects against myocardial I/RI by regulating lncRNA Gm4419-mediated epigenetic silencing of the DUSP5/ERK1/2 axis. Toxicol. Appl. Pharmacol. 433, 115782 (2021).
Article CAS PubMed Google Scholar
Wen, Z. P. et al. Knockdown ATG4C inhibits gliomas progression and promotes temozolomide chemosensitivity by suppressing autophagic flux. J. Exp. Clin. Cancer Res. 38(1), 298 (2019).
Article PubMed PubMed Central Google Scholar
Wu, C. et al. Genetic association, mRNA and protein expression analysis identify ATG4C as a susceptibility gene for Kashin-Beck disease. Osteoarthrx. Cartil. 25(2), 281–286 (2017).
Liu, P. et al. Epigallocatechin-3-gallate protects cardiomyocytes from hypoxia-reoxygenation damage via raising autophagy related 4C expression. Bioengineered 12(2), 9496–9506 (2021).
Article CAS PubMed PubMed Central Google Scholar
Kleinbongard, P., Andreadou, I. & Vilahur, G. The platelet paradox of injury versus protection in myocardial infarction-has it been overlooked?. Basic Res. Cardiol. 116(1), 37 (2021).
Article PubMed PubMed Central Google Scholar
Lieder, H.R. et al. Platelet-mediated transfer of cardioprotection by remote ischemic conditioning and its abrogation by aspirin but not by ticagrelor. Cardiovasc. Drugs Ther. (2022).
Lill, G. et al. Complex effects of different green tea catechins on human platelets. FEBS Lett. 546(2–3), 265–270 (2003).
Article CAS PubMed Google Scholar
Jin, Y. R. et al. Antiplatelet activity of epigallocatechin gallate is mediated by the inhibition of PLCgamma2 phosphorylation, elevation of PGD2 production, and maintaining calcium-ATPase activity. J. Cardiovasc. Pharmacol. 51(1), 45–54 (2008).
Article CAS PubMed Google Scholar
Joo, H. J. et al. Anti-platelet effects of epigallocatechin-3-gallate in addition to the concomitant aspirin, clopidogrel or ticagrelor treatment. Korean J. Intern. Med. 33(3), 522–531 (2018).
Article CAS PubMed Google Scholar
Lee, D. H. et al. Inhibitory effects of epigallocatechin-3-gallate on microsomal cyclooxygenase-1 activity in platelets. Biomol. Ther. (Seoul) 21(1), 54–59 (2013).
Article CAS PubMed Google Scholar
Millington-Burgess, S. L. & Harper, M. T. Epigallocatechin gallate inhibits release of extracellular vesicles from platelets without inhibiting phosphatidylserine exposure. Sci. Rep. 11(1), 17678 (2021).
Article ADS CAS PubMed PubMed Central Google Scholar
Hausenloy, D. J. et al. The coronary circulation in acute myocardial ischaemia/reperfusion injury: A target for cardioprotection. Cardiovasc. Res. 115(7), 1143–1155 (2019).
Article CAS PubMed Google Scholar
Heusch, G. Coronary microvascular obstruction: The new frontier in cardioprotection. Basic Res. Cardiol. 114(6), 45 (2019).
Heusch, G. Myocardial ischaemia–reperfusion injury and cardioprotection in perspective. Nat. Rev. Cardiol. 17(12), 773–789 (2020).
Dörge, H. et al. Coronary microembolization: The role of TNF-alpha in contractile dysfunction. J. Mol. Cell Cardiol. 34(1), 51–62 (2002).
Heusch, G. The coronary circulation as a target of cardioprotection. Circ. Res. 118(10), 1643–1658 (2016).
Article CAS PubMed Google Scholar
Liu, Y. et al. Protective effects and mechanism of curcumin on myocardial injury induced by coronary microembolization. J. Cell Biochem. 120(4), 5695–5703 (2019).
Article CAS PubMed Google Scholar
Saito, A. et al. The impact of theaflavins on systemic-and microcirculation alterations: The murine and randomized feasibility trials. J. Nutr. Biochem. 32, 107–114 (2016).
Article CAS PubMed Google Scholar
Widlansky, M. E. et al. Acute EGCG supplementation reverses endothelial dysfunction in patients with coronary artery disease. J. Am. Coll. Nutr. 26(2), 95–102 (2007).
Article CAS PubMed PubMed Central Google Scholar
Chatree, S. et al. Epigallocatechin gallate decreases plasma triglyceride, blood pressure, and serum kisspeptin in obese human subjects. Exp. Biol. Med. (Maywood) 246(2), 163–176 (2021).
Article CAS PubMed Google Scholar
Brown, A. L. et al. Effects of dietary supplementation with the green tea polyphenol epigallocatechin-3-gallate on insulin resistance and associated metabolic risk factors: Randomized controlled trial. Br. J. Nutr. 101(6), 886–894 (2009).
Article CAS PubMed Google Scholar
Suzuki-Sugihara, N. et al. Green tea catechins prevent low-density lipoprotein oxidation via their accumulation in low-density lipoprotein particles in humans. Nutr. Res. 36(1), 16–23 (2016).
Article CAS PubMed Google Scholar
Kurita, I. et al. Antihypertensive effect of Benifuuki tea containing O-methylated EGCG. J. Agric. Food Chem. 58(3), 1903–1908 (2010).
Article CAS PubMed Google Scholar
Kristen, A. V. et al. Green tea halts progression of cardiac transthyretin amyloidosis: An observational report. Clin. Res. Cardiol. 101(10), 805–813 (2012).
Article CAS PubMed PubMed Central Google Scholar
aus dem Siepen, F. et al. Extracellular remodeling in patients with wild-type amyloidosis consuming epigallocatechin-3-gallate: Preliminary results of T1 mapping by cardiac magnetic resonance imaging in a small single center study. Clin. Res. Cardiol. 104(8), 640–647 (2015).
Lorenz, M. et al. Tea-induced improvement of endothelial function in humans: No role for epigallocatechin gallate (EGCG). Sci. Rep. 7(1), 2279 (2017).
Article ADS PubMed PubMed Central Google Scholar
Widmer, R. J. et al. Beneficial effects of polyphenol-rich olive oil in patients with early atherosclerosis. Eur. J. Nutr. 52(3), 1223–1231 (2013).
Article CAS PubMed Google Scholar
Wu, M. Y. et al. Current mechanistic concepts in ischemia and reperfusion injury. Cell Physiol. Biochem. 46(4), 1650–1667 (2018).
Article CAS PubMed Google Scholar
Zeng, Y. F. et al. Cardioprotective effect of curcumin on myocardial ischemia/reperfusion injury: A meta-analysis of preclinical animal studies. Front. Pharmacol. 14, 1184292 (2023).
Article CAS PubMed PubMed Central Google Scholar
Sena, E. S. et al. Systematic reviews and meta-analysis of preclinical studies: Why perform them and how to appraise them critically. J. Cereb. Blood Flow Metab. 34(5), 737–742 (2014).
Article PubMed PubMed Central Google Scholar
Lecour, S. et al. IMproving Preclinical Assessment of Cardioprotective Therapies (IMPACT) criteria: Guidelines of the EU-CARDIOPROTECTION COST Action. Basic Res. Cardiol. 116(1), 52 (2021).
Article CAS PubMed PubMed Central Google Scholar
van der Worp, H. B. et al. Can animal models of disease reliably inform human studies?. PLoS Med. 7(3), e1000245 (2010).
Article PubMed PubMed Central Google Scholar
This study was supported by the National Natural Science Foundation of China (No.81903663), the Hunan Provincial Natural Science Foundation of China (No. 2020JJ5944), and Xiangya Hospital Central South University Postdoctoral Foundation.
These authors contributed equally: Xin-Yu Wei and Yi-Fan Zeng.
Department of Pharmacy, Xiangya Hospital, Central South University, Changsha, Hunan, China
Xin-Yu Wei, Qi-Hao Guo & Wen-Jing Zeng
National Clinical Research Center for Geriatric Disorders, Xiangya Hospital, Central South University, Changsha, China
Xin-Yu Wei & Wen-Jing Zeng
Department of Cardiovascular Surgery, The Second Xiangya Hospital, Central South University, Changsha, Hunan, China
Yi-Fan Zeng, Ji-Jia Liu & Ni Yin
Department of Pharmacy, Shengjing Hospital, China Medical University, Shenyang, China
Department of Pharmacy, Hunan Aerospace Hospital, Hunan Normal University, Changsha, Hunan, China
You can also search for this author in PubMed Google Scholar
You can also search for this author in PubMed Google Scholar
You can also search for this author in PubMed Google Scholar
You can also search for this author in PubMed Google Scholar
You can also search for this author in PubMed Google Scholar
You can also search for this author in PubMed Google Scholar
You can also search for this author in PubMed Google Scholar
Y.Z.: Conceptualization, Methodology, Formal analysis. X.W.: Writing-original draft. Q.G.: Data curation. J.L.: Visualization, Investigation. N.Y.: Supervision. Y.L.: Writing- Reviewing and Editing. W.Z.: Funding acquisition, Writing- Reviewing and Editing. All authors reviewed the manuscript.
The authors declare no competing interests.
Springer Nature remains neutral with regard to jurisdictional claims in published maps and institutional affiliations.
Open Access This article is licensed under a Creative Commons Attribution 4.0 International License, which permits use, sharing, adaptation, distribution and reproduction in any medium or format, as long as you give appropriate credit to the original author(s) and the source, provide a link to the Creative Commons licence, and indicate if changes were made. The images or other third party material in this article are included in the article's Creative Commons licence, unless indicated otherwise in a credit line to the material. If material is not included in the article's Creative Commons licence and your intended use is not permitted by statutory regulation or exceeds the permitted use, you will need to obtain permission directly from the copyright holder. To view a copy of this licence, visit http://creativecommons.org/licenses/by/4.0/.
Wei, XY., Zeng, YF., Guo, QH. et al. Cardioprotective effect of epigallocatechin gallate in myocardial ischemia/reperfusion injury and myocardial infarction: a meta-analysis in preclinical animal studies. Sci Rep 13, 14050 (2023). https://doi.org/10.1038/s41598-023-41275-2
DOI: https://doi.org/10.1038/s41598-023-41275-2
Anyone you share the following link with will be able to read this content:
Sorry, a shareable link is not currently available for this article.
Provided by the Springer Nature SharedIt content-sharing initiative
By submitting a comment you agree to abide by our Terms and Community Guidelines. If you find something abusive or that does not comply with our terms or guidelines please flag it as inappropriate.
Scientific Reports (Sci Rep) ISSN 2045-2322 (online)
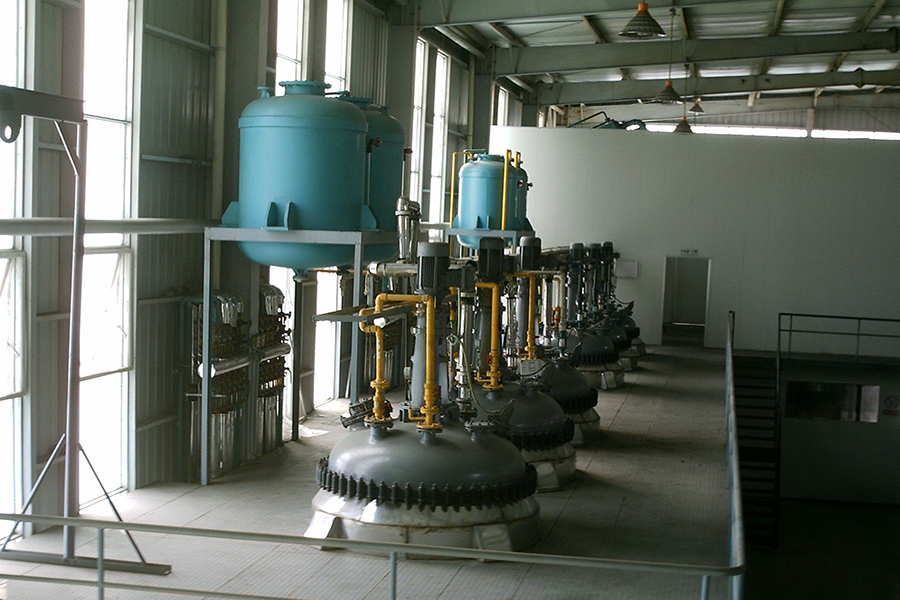
Gallic Acid Powder Sign up for the Nature Briefing newsletter — what matters in science, free to your inbox daily.